Learn how steel bracing systems provide lateral stability for structures, resisting wind and seismic forces with effective design for overall safety and strength.
Introduction
Selecting and designing a lateral force restraining system for a steel structure has significant implications on its overall stability. As well as resisting vertical loadings, all structures must resist lateral loading as a result of wind or earthquakes which are simplified into a static lateral load for design.
Whether it be a tall tower resisting seismic loading, a bay of a stadium resisting torsion from cantilever seating, or even a humble shed resisting the wind, all structures need a lateral stability system.

For most large buildings, the primary lateral resistance system is a "core", typically made up of a series of RC shear wallsenclosing elevators and stairs. In some cases, the core alone is unable to resist the immense loads caused by wind and earthquakes. One method to increase the lateral stiffness, which is the ability to resist lateral deflection, of a building is to incorporate steel bracing systems.

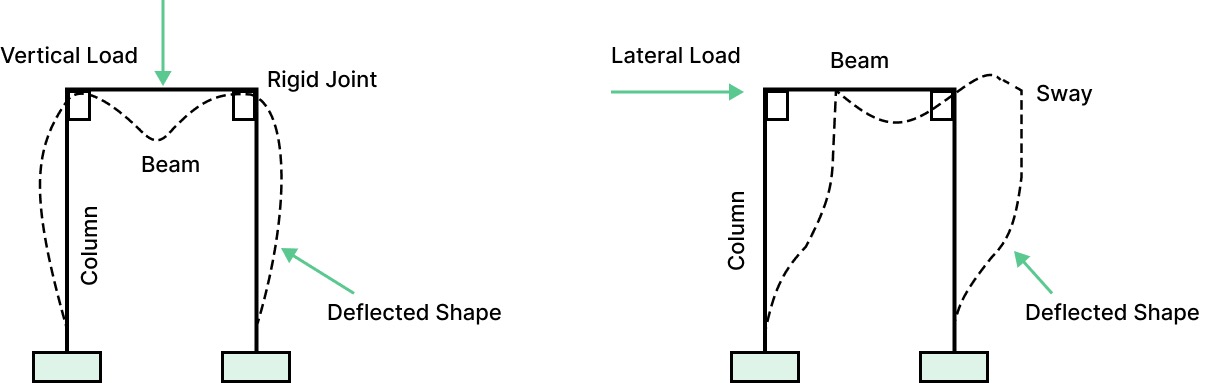
Tension members are preferred for bracing elements since compression members can be subject to buckling (and therefore the steel section may need to be bigger). Bracing elements are often placed at 45⁰ to achieve a beam-column juncture. Inclinations less than 45⁰ will increase the sway sensitivity of the structure.

In this article, we go through the common types of steel-braced frame systems and provide a step-by-step guide on how to design them. Braced frames are mainly suitable for low-rise to mid-rise buildings since they are not incredibly effective in resisting torsional loads compared to shear walls.
The primary objectives when designing steel-braced frames are to:
- Direct lateral load in horizontal beams and diaphragms to angled struts. Thereby leveraging the axial strength of brace members and providing load paths transferring the lateral load into vertical elements and down to foundations.
- Reduce deflection and sway caused by lateral loading by stiffening parts of the structure. This could be done with a roof diaphragm, a belt and braces outrigger or a vertical wall. Stiffening the structure also defines a clear load path along where you as the designer want the load to go, e.g. stiffening a roof plane with steel bracing and adding vertical brace bays, provides a clear load path of lateral roof loads into the brace bays and down to the foundations.
- Control global buckling and torsion by providing steel bracing systems on at least three of the four sides of a building. For local buckling and torsion of the steel bracing, implement a direct lateral restraint for the compression flanges or torsional restraint to the whole beam.
💡What are Diaphragms?
They are structural elements that can transfer lateral loads to the vertical resisting elements of a structure (i.e. shear walls and columns) primarily through in-plane shear stress. They are typically horizontal and are formed in roofs and floors.
\( \bf{1}\) Applications of Steel Bracing
In all structures, lateral resistance is required in the X (horizontal) and Y (vertical) primary planes. This is because lateral load must be taken by horizontal structural elements (a diaphragm) to then be transferred to vertical structural elements, so that the load can make it's way down to the foundations. Here, we will discuss vertical and horizontal bracing systems.
Vertical Bracing Systems

Vertical bracing bays consist of angled steel strut and/or tie members that span diagonally between orthogonal beams and columns. They are typically positioned in vertical planes, between lines of columns or within partition walls. In buildings, they provide load paths for lateral forces generated in floor and roof diaphragms undergoing seismic and wind actions down to the foundations. They work to transform horizontal loads to vertical ‘push-pull’ loads in primary column groups through axial loads in the diagonal bracing elements.
Depending on the structure, brace bays will be used in conjunction with other lateral stability elements, like cores, moment frames or shear walls.
Structurally, vertical bays are ideally placed at the extremities of the structure in order to resist global torsion. Architecturally, vertical bays are often placed within partition walls or otherwise so they can be hidden.

Horizontal Bracing Systems

Horizontal steel bracings can be used to form flat or angled diaphragms, typically in floor and roof systems. Here the bracing works to stiffen the floor or roof and hence creates a diaphragm. This stiffness increase provides an effective path for lateral loads to travel to vertically stiff elements.
In buildings, the structure that forms floors and roofs has an inherent lateral resistance which is often stiff enough to establish a diaphragm. Nonetheless, in situations where the floor or roof doesn't have required lateral stiffness, steel bracing can be added. They are often referred to as an "exo-cross-brace" and are diagonal tension/compression members positioned beneath the floor and roof.
For roofs, horizontal bracing is typically cross-ties (tension-only rod elements arranged in an X pattern bay to bay). This is because lateral loads generated at the roof plane are notably less pronounced compared to those imposed on the structural floor plane. Restricting loading exclusively to tension is achieved by using ties and flat plates, which are components that poss either negligible or no compressive capacity.

\( \bf{2}\) Types of Braces
Concentric Braced Frames
Concentric bracing involves diagonal braces positioned within the frame's plane. Both ends of the brace connect at the end point of other framing members, culminating in the formation of a truss-like structure to build a stiff frame. While concentric bracing is efficient in resisting lateral forces, it can be susceptible to buckling under large loads.

Eccentric Braced Frames
Like concentric bracing, eccentric bracing is arranged in a way that diagonal bracing is positioned in the plane of the frame. The primary difference you may have noticed is that they do not connect at the endpoints of another framing member. The eccentricity of the brace connection helps prevent buckling under large loads since their effective length is reduced. Eccentric bracing can undergo large deformations without collapsing compared to concentric bracing.

\( \bf{3}\) Steps for Designing Steel Bracing - General Guide
Step 1. Select an Appropriate Bracing Type
To start, identify the loading conditions imposed on the structure. This could include:
- Dead loads
- Live loads
- Wind loads
- Seismic loads
- Temperature effects etc.
Select the appropriate bracing type based on the structure and loading conditions and determine their location in the structure. Consider the general layout and arrangement of the bracing elements.
Step 2. Calculate Design Loads
Calculate the design loads and determine the resulting forces and moments in the bracing members.
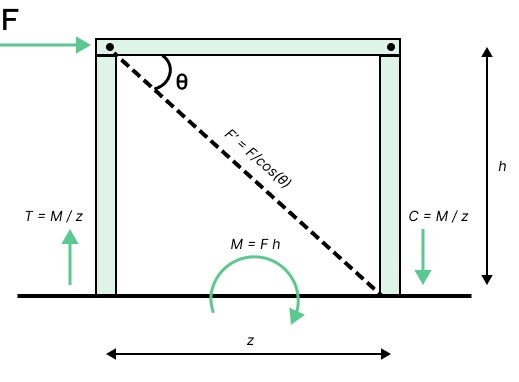
Step 3. Select Bracing Member Section
Select suitable steel sections for the first design reiteration of the bracing members.
Some steel sections that are often used in braced frames:
- I-Sections or W-Sections (Wide Flange Beams): Ability to resist both bending and shear forces.
- Angle Sections: L-shaped cross sections commonly used for diagonal bracing members.
- Channel Sections: C-shaped cross sections.
Some links to relevant steel sections catalogues:
- One Steel - Hot Rolled and Structural Steel products (Australia)
- Steel Building Design: Design Data SCI-363 (Eurocode)
- AISC Steel Construction Manual Design Tables (US)
Step 4. Check Axial Capacity & Compression Buckling Resistance
For tension members, design the members for axial tension. Some relevant design standards for designing for tension and compression members:
AS 4100: Steel Structures Clause 6.3 & 7.2 (Australia)
Check out our step-by-step design guide to Australian Standards here
BS EN 1993-1-1:2005 Design of Steel Structures Clause 6.3.3 (Eurocode)



ANSI/AISC 360-16: Structural Steel Buildings Chapter D & E (USA)
Chapter D - Design of Members for Tension
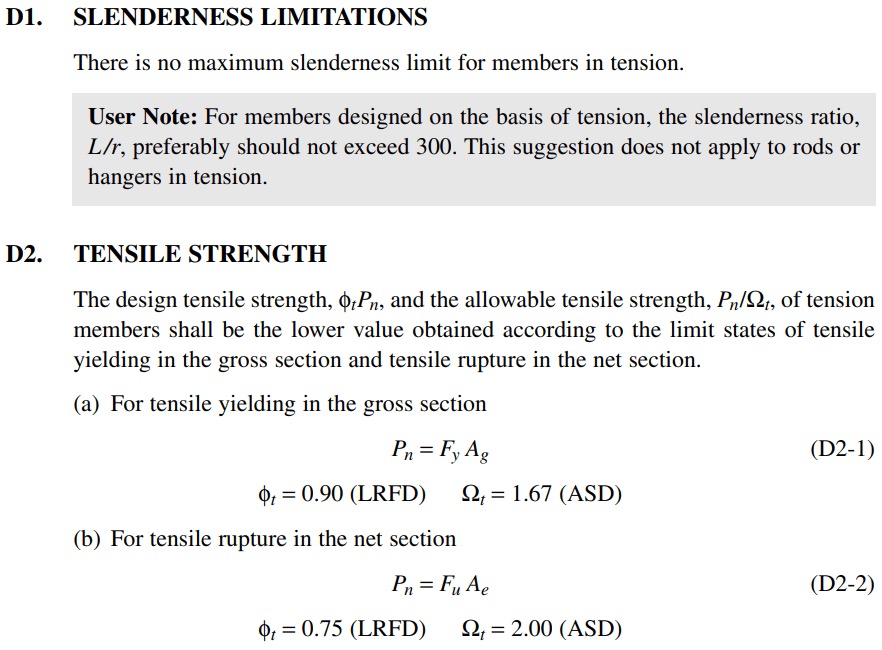

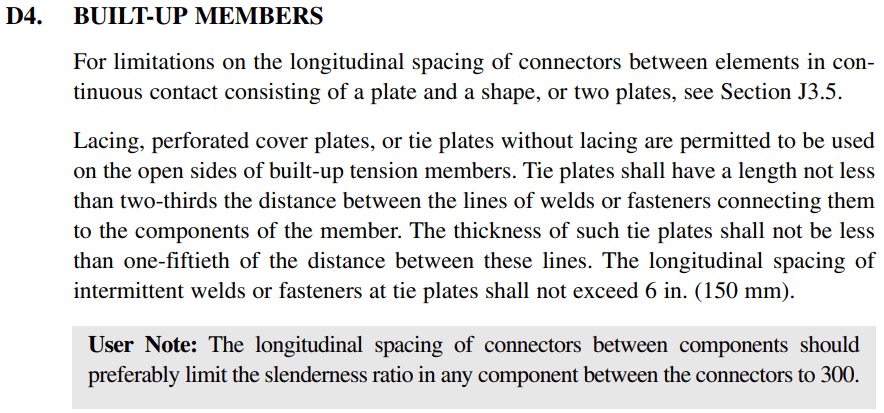



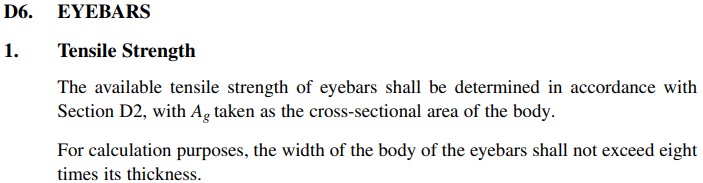
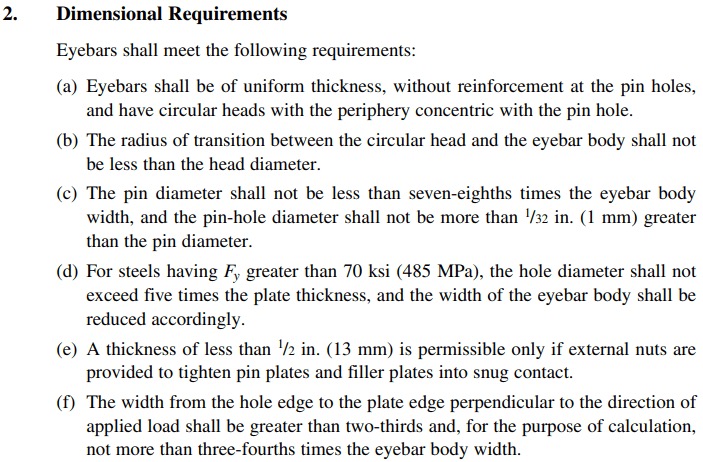
Chapter E - Design of Members for Compression
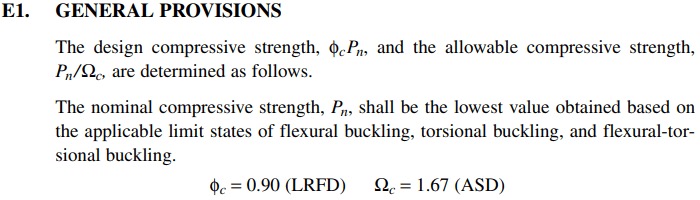

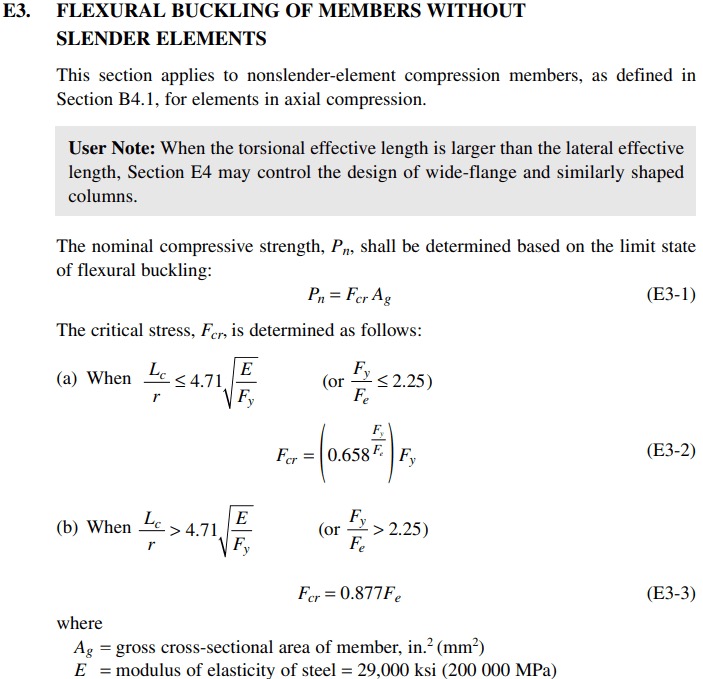
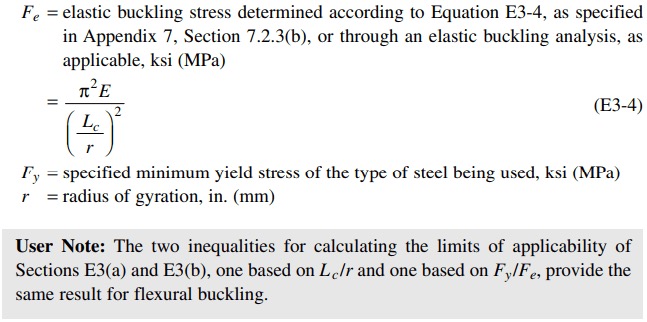

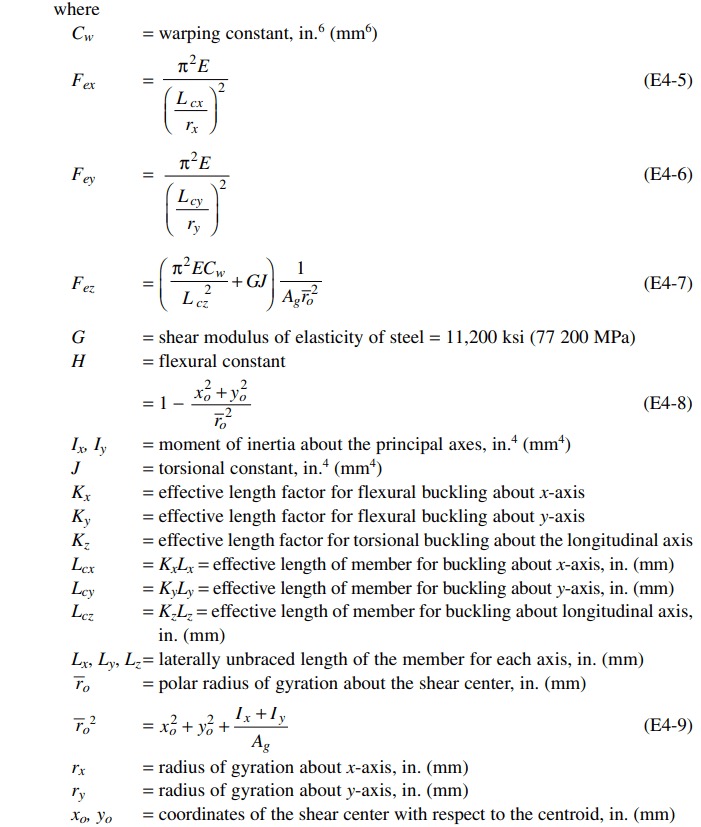


In general, members in compression are more complex to design since they can fail under:
- Yielding
- Inelastic buckling
- Elastic buckling (depending on the slenderness ratio)
Evaluate the frame stability and determine its sensitivity to second-order effects, then select an amplification factor for the horizontal load.
Step 5. Design Connections
As they say, a chain is only as strong as its weakest link. There's no point in creating a steel bracing system without adequate connections.
Of course, the design capacity of each connection element should not be less than the calculated design action effects.
Some relevant design standards for designing for tension and compression members:
AS 4100: Steel Structures Section 9 (Australia)
Check out our step-by-step design guide to Australian Standards here
BS EN 1993-1-8:2005 Design of Joints (Eurocode)
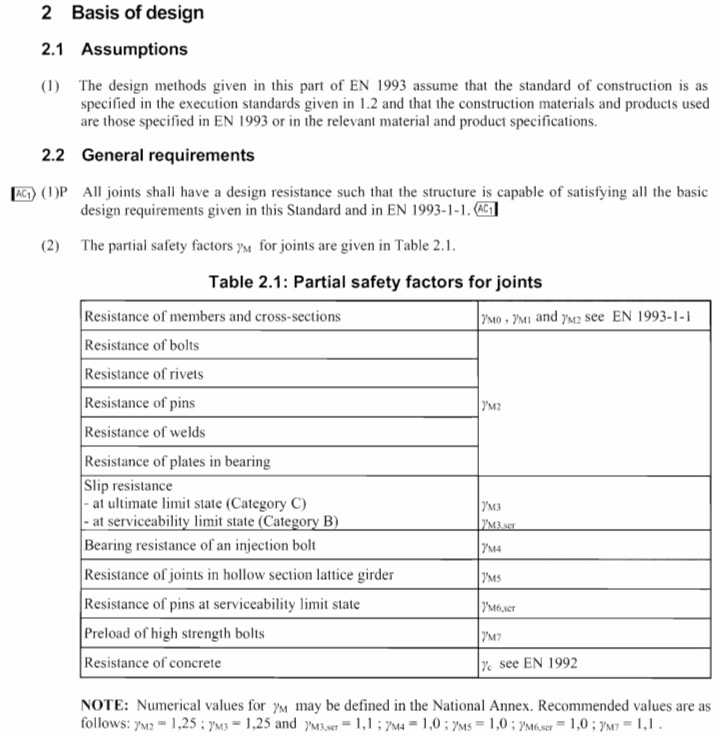
ANSI/AISC 360-16: Structural Steel Buildings Chapter J (USA)
